
A star is a massive, luminous ball of plasma that is held together by gravity. The nearest star to Earth is the Sun, which is the source of most of the energy on Earth. Other stars are visible in the night sky, when they are not outshone by the Sun. Historically, the most prominent stars on the celestial sphere were grouped together into constellations, and the brightest stars gained proper names. Extensive catalogues of stars have been assembled by astronomers, which provide standardized star designations.
For most of its life, a star shines due to thermonuclear fusion in its core releasing energy that traverses the star's interior and then radiates into outer space. Almost all naturally occurring elements heavier than helium were created by stars, either via stellar nucleosynthesis during their lifetimes or by supernova nucleosynthesis when stars explode. Astronomers can determine the mass, age, chemical composition and many other properties of a star by observing its spectrum, luminosity and motion through space. The total mass of a star is the principal determinant in its evolution and eventual fate. Other characteristics of a star are determined by its evolutionary history, including diameter, rotation, movement and temperature. A plot of the temperature of many stars against their luminosities, known as a Hertzsprung-Russell diagram (H–R diagram), allows the age and evolutionary state of a star to be determined.

The Hertzsprung -Russell (H-R) Diagram is a graph that plots stars color (spectral type or
A star begins as a collapsing cloud of material composed primarily of hydrogen, along with helium and trace amounts of heavier elements. Once the stellar core is sufficiently dense, some of the hydrogen is steadily converted into helium through the process of nuclear fusion. The remainder of the star's interior carries energy away from the core through a combination of radiative and convective processes. The star's internal pressure prevents it from collapsing further under its own gravity. Once the hydrogen fuel at the core is exhausted, those stars having at least 0.4 times the mass of the Sun expand to become a red giant, in some cases fusing heavier elements at the core or in shells around the core. The star then evolves into a degenerate form, recycling a portion of the matter into the interstellar environment, where it will form a new generation of stars with a higher proportion of heavy elements.


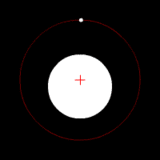

Binary and multi-star systems consist of two or more stars that are gravitationally bound, and generally move around each other in stable orbits. When two such stars have a relatively close orbit, their gravitational interaction can have a significant impact on their evolution. Stars can form part of a much larger gravitationally bound structure, such as a cluster or a galaxy.

STELLAR CLASSIFICATION
Stellar classification is a classification of stars based on their spectral characteristics. The spectral class of a star is a designated class of a star describing the ionization of its chromosphere, what atomic excitations are most prominent in the light, giving an objective measure of the temperature in this chromosphere.
Most stars are currently classified using the letters O, B, A, F, G, K and M, where O stars are the hottest and the letter sequence indicates successively cooler stars up to the coolest M class. According to an informal tradition, O stars are "blue", B "blue-white", A stars "white", F stars "yellow-white", G stars "yellow", K stars "orange", and M stars "red", even though the actual star colors perceived by an observer may deviate from these colors depending on visual conditions and individual stars observed.

The Harvard classification system is a one-dimensional classification scheme. Stars vary in surface temperature from about 2 to 40 kK (2,000 to 40,000 kelvins). Physically, the classes indicate the temperature of the star's atmosphere and are normally listed from hottest to coldest, as is done in the following table
Class | Temperature[8] (kelvins) | Conventional color | Mass[8] (solar masses) | Radius[8] (solar radii) | Luminosity[8] (bolometric) | Fraction of all main sequence stars[12] |
---|---|---|---|---|---|---|
O | ≥ 33,000 K | blue | ≥ 16 M☉ | ≥ 6.6 R☉ | ≥ 30,000 L☉ | ~0.00003% |
B | 10,000–30,000 K | blue to blue white | 2.1–16 M☉ | 1.8–6.6 R☉ | 25–30,000 L☉ | 0.13% |
A | 7,500–10,000 K | white | 1.4–2.1 M☉ | 1.4–1.8 R☉ | 5–25 L☉ | 0.6% |
F | 6,000–7,500 K | yellowish white | 1.04–1.4 M☉ | 1.15–1.4 R☉ | 1.5–5 L☉ | 3% |
G | 5,200–6,000 K | yellow | 0.8–1.04 M☉ | 0.96–1.15 R☉ | 0.6–1.5 L☉ | 7.6% |
K | 3,700–5,200 K | orange | 0.45–0.8 M☉ | 0.7–0.96 R☉ | 0.08–0.6 L☉ | 12.1% |
M | ≤ 3,700 K | red | ≤ 0.45 M☉ | ≤ 0.7 R☉ | ≤ 0.08 L☉ | 76.45% |
LIFE CYCLE OF THE SUN AND SOLAR SYSTEM
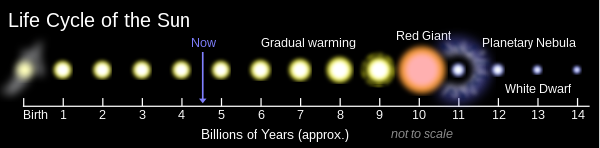
The time frame of the Solar System's formation has been determined using radiometric dating. Scientists estimate that the Solar System is 4.6 billion years old. The oldest known mineral grains on Earth are approximately 4.4 billion years old. Rocks this old are rare, as Earth's surface is constantly being reshaped by erosion, volcanism, and plate tectonics. To estimate the age of the Solar System, scientists use meteorites, which were formed during the early condensation of the solar nebula. Almost all meteorites (see the Canyon Diablo meteorite) are found to have an age of 4.6 billion years, suggesting that the Solar System must be at least this old. Studies of discs around other stars have also done much to establish a time frame for Solar System formation. Stars between one and three million years old possess discs rich in gas, whereas discs around stars more than 10 million years old have little to no gas, suggesting that gas giant planets within them have ceased forming.
PLANETARY SYSTEM
A planetary system consists of the various non-stellar objects orbiting a star such as planets, dwarf planets, moons, asteroids, meteoroids, comets, and cosmic dust. The Sun together with its planetary system, which includes Earth, is known as the Solar System.

Planetary systems are generally believed to form as part of the same process which results in star formation. Some early theories involved another star passing extremely close to the Sun, drawing material out from it which then coalesced to form the planets.
BIRTH OF STAR - THE EAGLE NEBULA
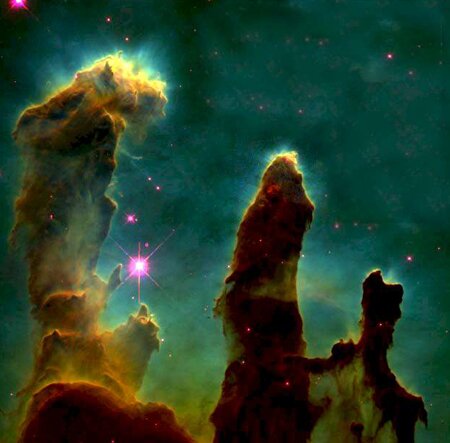
This part of the Eagle Nebula once looked like any other wispy cloudy nebula. But at some point in the past, new massive hot stars located above the frame of the photo started blazing away. They now send out intense ultraviolet radiation that sweeps away the gas and dust that makes up the nebula. It also heats up parts of the nebula enough to make them glow.
Why did the pillars form? The head of each pillar contains exceptionally dense clots of gas and dust that represent baby stars in the process of formation. Their strong gravitational fields pull in the surrounding gas and dust and thus hold this material in place against the stellar winds. The parts of the nebula 'downwind' of the clusters of baby stars are shielded from the wind and thus form pillars.
The baby stars need to reach a certain size before their centers attain the high temperatures and pressures needed to start up the nuclear fusion reactions that make a star a star. The baby stars in the heads of these columns are in a race against time: they have to suck in enough material to reach the right size before the rest of the surrounding gasses are blasted away. If they don't grow large enough, they won't become a proper star.
The longest pillar is 1 light-year long (about 5.88 trillion miles, or about 800 times longer than the diameter of our Solar System). The clouds consist mostly of hydrogen with traces of other gasses and dust. The microscopic dust particles include graphite and certain rocks and minerals found on Earth.
CLOSE-UP OF THE EAGLE NEBULA

The tip of this column shows a number of 'fingers' pointing to the upper right. Each finger hosts in its tip a baby star. Some of these protostars may never ignite because they will be too small by the time the stellar winds strip away the raw materials needed for growth. You can see a tiny, faint, cocoon-shaped mass just to the upper-left of the pillar; this is a baby that has been cut off, apparently before it could ignite its nuclear furnace. In the upper right is a finger that is almost detached from the main pillar.
STAR FORMATION
Star formation is the process by which dense parts of molecular clouds collapse into a ball of plasma to form a star. As a branch of astronomy star formation includes the study of the interstellar medium and giant molecular clouds (GMC) as precursors to the star formation process and the study of young stellar objects and planet formation as its immediate products. Star formation theory, as well as accounting for the formation of a single star, must also account for the statistics of binary stars and the initial mass function.


Planetary systems are generally believed to form as part of the same process which results in star formation. Some early theories involved another star passing extremely close to the Sun, drawing material out from it which then coalesced to form the planets. However, the probability of such a near collision is now known to be far too low to make this a viable model. Accepted theories today argue that a protoplanetary disk forms by gravitational collapse of a molecular cloud and then evolves into a planetary system by collisions and gravitational capture.
Some planetary systems may form differently, however. Planets orbiting pulsars—stars which emit periodic bursts of electromagnetic radiation—have been discovered by the slight variations they cause in the timing of these bursts. Pulsars are formed in violent supernova explosions, and a normal planetary system could not possibly survive such a blast—planets would either evaporate, be pushed off of their orbits by the masses of gas from the exploding star, or the sudden loss of most of the mass of the central star would see them escape the gravitational hold of the star. One theory is that existing stellar companions were almost entirely evaporated by the supernova blast, leaving behind planet-sized bodies. Alternatively, planets may somehow form in the accretion disk surrounding pulsars.
SUPERNOVA INDUCED STAR FORMATION

The very massive stars form first and explode into supernova. This makes shock waves into the molecular cloud, causing nearby gas to compress and form more stars. This allows a type of stellar coherence (young stars are found near other young stars) to build up, and is responsible for the pinwheel patterns we see in galaxies.
LOW MASS AND HIGH MASS STAR
Difference between low-mass star and high-mass star.

Stars of different masses are thought to form by slightly different mechanisms. The theory of low-mass star formation, which is well-supported by a plethora of observations, suggests that low-mass stars form by the gravitational collapse of rotating density enhancements within molecular clouds. As described above, the collapse of a rotating cloud of gas and dust leads to the formation of an accretion disk through which matter is channeled onto a central protostar. For stars with masses higher than about 8 solar masses, however, the mechanism of star formation is not well understood.
Massive stars emit copious quantities of radiation which pushes against infalling material. In the past, it was thought that this radiation pressure might be substantial enough to halt accretion onto the massive protostar and prevent the formation of stars with masses more than a few tens of solar masses. Recent theoretical work has shown that the production of a jet and outflow clears a cavity through which much of the radiation from a massive protostar can escape without hindering accretion through the disk and onto the protostar. Present thinking is that massive stars may therefore be able to form by a mechanism similar to that by which low mass stars form.
No comments:
Post a Comment
Note: Only a member of this blog may post a comment.